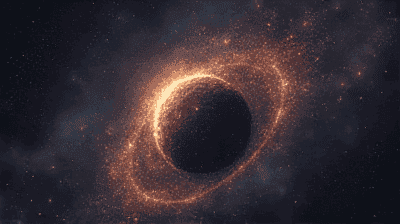
In the vast cosmos, a mysterious substance eludes direct detection while profoundly influencing the structure and evolution of the universe. This elusive entity is known as dark matter, a form of matter that does not emit, absorb, or reflect light, making it invisible to traditional observational techniques. Despite its ghostly presence, dark matter is believed to constitute approximately 27 percent of the universe's total mass-energy content. Understanding dark matter is one of the foremost challenges in modern astrophysics and cosmology.
Understanding Dark Matter
What is Dark Matter?
Dark matter is a theoretical form of matter that was introduced to explain discrepancies between observed galaxy rotation curves and the predictions made by Newtonian dynamics and general relativity. Unlike ordinary matter, which makes up stars, planets, and visible galaxies, dark matter does not interact with electromagnetic forces, rendering it invisible. Thus, it can only be inferred through its gravitational effects on visible matter and radiation.
Why Do We Believe in Dark Matter?
The belief in dark matter arises from several lines of evidence, including:
Galaxy Rotation Curves: Observations of spiral galaxies reveal that stars at the outer edges of these galaxies rotate at speeds that cannot be accounted for by the visible mass alone. According to Newtonian gravity, the outer stars should rotate more slowly. The presence of dark matter provides the additional gravitational pull needed to explain these observed speeds.
Gravitational Lensing: Dark matter can bend light from distant galaxies through a phenomenon known as gravitational lensing. When massive objects, such as galaxy clusters, lie between a distant light source and an observer, the light's path is distorted. The degree of lensing reveals the presence and distribution of dark matter.
Cosmic Microwave Background: Studies of the Cosmic Microwave Background radiation provide insights into the early universe's conditions and confirm the presence of dark matter through the analysis of temperature fluctuations.
Large Scale Structure: The formation of galaxies and clusters on cosmic scales is influenced by dark matter. Simulations of cosmic structure formation that include dark matter align more closely with observed large-scale structures than those that consider only visible matter.
Types of Dark Matter
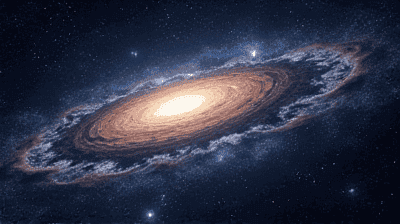
Weakly Interacting Massive Particles (WIMPs)
WIMPs are among the leading candidates for dark matter. These particles are predicted by various theoretical frameworks, including supersymmetry. WIMPs would interact with ordinary matter via the weak nuclear force and gravity, making them challenging to detect.
Axions
Axions are hypothetical particles that could solve several theoretical problems in particle physics, including the strong CP problem. They are very light and would interact with matter through extremely weak forces. Axions could form a "sea" of particles throughout the universe.
Sterile Neutrinos
Sterile neutrinos are another type of dark matter candidate that differs from the known neutrinos. Unlike ordinary neutrinos, sterile neutrinos do not interact via the standard weak interactions, making them much harder to detect.
Modified Gravity Theories
While dark matter exists as a substance, some alternative theories propose modifications to the laws of gravitation to explain the observed phenomena without invoking dark matter directly. These modifications, though less popular, are still subjects of investigation.
The Importance of the Dark Matter Hunt
Understanding the Universe's Composition
Searching for dark matter is crucial for elucidating the universe's composition. With only about 5 percent of the universe comprising ordinary matter, understanding dark matter may reveal insights into the fundamental nature of the cosmos.
Probing Fundamental Physics
The hunt for dark matter also probes the boundaries of particle physics. Detecting dark matter particles could provide tests of theories beyond the Standard Model, including supersymmetry and extra dimensions.
Cosmological Implications
Understanding dark matter is essential for constructing comprehensive models of cosmic evolution. Dark matter influences galaxy formation, structure, and dynamics, and determining its properties will enhance our understanding of the universe's history.
Cutting Edge Experiments in the Dark Matter Hunt
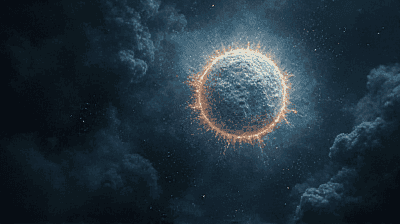
Numerous experiments are underway across the globe, employing innovative techniques and technologies in the quest to catch dark matter. Here are some of the notable endeavors.
1. Direct Detection Experiments
Direct detection experiments aim to observe dark matter particles interacting with ordinary matter. These experiments typically involve sensitive detectors housed deep underground to shield them from cosmic rays and other noise.
a. LUX-ZEPLIN (LZ)
The LUX-ZEPLIN experiment is a state-of-the-art dark matter detection experiment located at the Sanford Underground Research Facility in South Dakota. It employs a dual-phase xenon time projection chamber to capture potential interactions between WIMPs and xenon nuclei. The experiment aims to achieve unprecedented sensitivity to dark matter, operating with nearly 10 tons of liquid xenon.
b. XENONnT
Located at the Gran Sasso National Laboratory in Italy, the XENONnT experiment is an upgrade to the XENON1T experiment. It uses liquid xenon as its target material and features advanced technologies for detecting tiny signals arising from potential dark matter interactions. XENONnT is designed to improve sensitivity and reduce background noise, enhancing the likelihood of successful detection.
c. PandaX
The PandaX experiment is located in the Jinping Underground Laboratory in China. It employs a similar dual-phase liquid xenon detector approach, with a focus on exploring the parameter space of WIMPs. The collaboration aims to analyze data from thousands of kilograms of liquid xenon to search for elusive dark matter signals.
2. Indirect Detection Experiments
Indirect detection experiments search for products of dark matter annihilation or decay, such as gamma rays, neutrinos, or cosmic rays. By observing high-energy particles, scientists hope to infer the presence and properties of dark matter.
a. Fermi Gamma-ray Space Telescope
The Fermi Gamma-ray Space Telescope has been instrumental in observing the gamma-ray sky. Scientists analyze gamma-ray emissions from regions with expected high dark matter density, such as the centers of galaxies or regions of galaxy clusters. Anomalies in gamma-ray spectra could indicate dark matter interactions.
b. IceCube Neutrino Observatory
Situated at the South Pole, the IceCube Neutrino Observatory detects high-energy neutrinos produced by cosmic processes. In addition to studying astrophysical sources, IceCube may detect neutrinos resulting from dark matter interactions or annihilation, providing essential clues about dark matter properties.
c. AMS-02 (Alpha Magnetic Spectrometer)
The Alpha Magnetic Spectrometer is a particle physics experiment mounted on the International Space Station. AMS-02 detects cosmic rays and looks for potential signals of dark matter through anomalies in cosmic ray spectra. The quest for unusual positron and antiproton ratios has drawn particular attention.
3. Collider Experiments
High-energy particle colliders, such as CERN's Large Hadron Collider (LHC), also contribute to the dark matter hunt by searching for dark matter candidates in high-energy collisions.
a. Large Hadron Collider (LHC)
The LHC accelerates protons to nearly the speed of light, producing a variety of particles when collisions occur. While its primary objective is to search for the Higgs boson and explore new physics beyond the Standard Model, the LHC is also a crucial tool for investigating potential dark matter signals. Particle collisions may produce WIMPs or other exotic particles that could hint at dark matter.
4. Astrophysical Observations
Astrophysical observations across various wavelengths provide valuable insights into dark matter's influence on cosmic structures.
a. Galaxy Surveys
Large-scale galaxy surveys, such as the Sloan Digital Sky Survey (SDSS) and the European Space Agency's Euclid mission, map the distribution of galaxies and galaxy clusters in the universe. These observations can provide constraints on dark matter’s collective effects and contribute to understanding cosmic structure.
b. Gravitational Lensing Studies
Gravitational lensing offers a way to infer dark matter distribution by analyzing the bending of light from distant objects due to the gravitational influence of foreground masses. Studies of galaxy clusters using gravitational lensing provide insights into the amount and distribution of dark matter in these regions.
Challenges in the Dark Matter Hunt
The Sensitivity of Detection
One of the primary challenges in identifying dark matter is its incredibly weak interaction with ordinary matter. As a result, the chances of detecting dark matter signals are extremely low, requiring highly sensitive detectors and long observation periods.
Background Noise
Ambient radiation from cosmic rays, radioactive isotopes, and other sources can obscure potential dark matter signals. Experiments are often conducted deep underground or in shielded environments to minimize background noise and enhance detection sensitivity.
Theoretical Uncertainties
The nature of dark matter and its underlying properties remain uncertain. Various candidates possess different interaction mechanisms and mass ranges, complicating the design and interpretation of experiments. Researchers must continue refining theories and models to optimize their search strategies.
Future Directions in Dark Matter Research
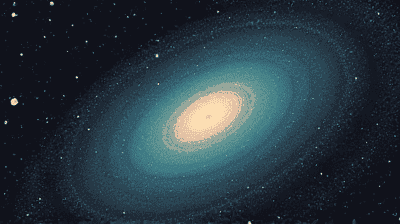
New Technologies and Innovations
The future of dark matter research relies on continued technological advances in detector designs, data analysis techniques, and theoretical modeling:
Cryogenic Detectors: Advances in cryogenic technologies aim to develop ultra-sensitive detectors that operate at near absolute zero temperatures, enhancing capabilities for seeking rare dark matter interactions.
Machine Learning Techniques: The application of machine learning and data science techniques can improve the analysis of vast datasets generated by experiments, enabling scientists to identify potential signals more efficiently.
Space-Based Observations: Future space-based missions designed to study cosmic phenomena could complement ground-based efforts by providing unique perspectives on dark matter through astrophysical observations.
Collaborative Efforts
Collaboration across disciplines and institutions will be critical for addressing the complexities of dark matter research. Partnerships between astrophysicists, particle physicists, and cosmologists will lead to a more comprehensive understanding of dark matter.
Broader Implications of Discovery
If dark matter is eventually detected, the discovery will have profound implications not only for cosmology and physics but also for our understanding of the universe. New physics beyond the Standard Model could be revealed, prompting reevaluation of fundamental theories governing particles and forces.
Conclusion
The hunt for dark matter is one of the most exciting endeavors in modern science, bridging the disciplines of astrophysics, particle physics, and cosmology. As scientists employ cutting-edge experiments and technologies in their quest to capture the universe's ghost, they inch closer to unraveling the mysteries of dark matter.
With ongoing advancements, the next decade promises to be pivotal for dark matter research. Whether through direct detection, indirect signals, collider experiments, or astrophysical observations, the quest to understand dark matter will lead to profound revelations about the universe and our place within it. By unraveling the secrets of dark matter, we move closer to deciphering the very fabric of reality itself.